3D Bioprinters – The Modular Solution for Tissue Engineering from GeSiM
Additive manufacturing (AM) for tissue engineering (TE) and regenerative medicine combines biocompatible/biodegradable polymers with living cells. GeSiM 3D bioprinters can create bioscaffolds for cell growth or deposit layers of bioinks on implants or microfluidic objects. However, artificial bones, skin, organoids still not reach their originals made by nature. Research on this subject requires flexible lab equipment, compatible even with new tools and accessories still to be developed.
Our bioprinters offer this level of versatility. Pneumatic extrusion is on board of each GeSiM bioprinter, numerous tools can be configured with your order or added years later. The user interface fits into this philosophy and adapts to each individual combination of tools.
Most extrusion tools fit to all GeSiM instruments. The combination of 3D printed scaffolds with Nanolitre pipetting and microfluidics extends the field of TE to drug discovery, cell biology and more.
Applications at a Glance:
- 3D Printing of very different (Bio-)polymers (can contain cells)
- Design and print of porous – multi material – structures for tissue engineering
- Co-printing of hard (E.g. PCL/PLA) and soft (E.g. Alginate) biopolymers (Sequential printing, co-axial extrusion, Nanolitre pipetting)
- Functionalization of metal implants with soft polymer layers
- Printing of micro structures from biopolymers by melt electro spinning/writing
- Co-printing of two polymer melts through a gradient mixer
Don’t miss the tab CUSTOMER REFERENCES outlining selected customer projects.
Instruments
BS3.3 Prime
The choice for beginners with potentially growing demands.
It is an attractively priced but complete bioprinter package:
- One temperature-controlled print head,
- LED UV-light, different wave lengths available,
- Pneumatic and mechanical extrusion,
- Syringe volumes from 10…50 Millilitres.
It prints a wide range of Hydrogels, Alginates and low-melting polymers like certain PCLs.
BS3.3
BS3.3 and it’s predecessors are in use for years in labs which paved the way for tissue engineering.
It is an individually configurable bioprinter for cutting-edge research. The range of available extrusion tools accepts almost any polymer of potential use in the field of TE. It combines highest flexibility with compact dimensions and fits in many popular biosafety cabinets.
BS5.3; BS5.3/CB
It’s the perfect companion for large research teams, core facilities and service providers with 24/7 operation.
BS5.3 comes with extremely powerful linear motors. This bioprinter can run unrivalled systems for gradient mixing of thermoplastic biopolymers during the print, in addition to all other extrusion tools.
BS5.3/CB is installed in a ground born GeSiM clean bench. This bench features a HEPA filter, a safety lock and a status indicator light on the roof (Picture on bottom of the page).
BS5.3/E
The largest GeSiM bioprinter tops our portfolio.
BS5.3/E offers almost double print bed size than all other bioprinters. The X/Y printing range is probably the largest on the market of commercial bioprinters. Customized object holders for batch production are available, please ask.
Smart Technology for Efficient Use
- Silent but smart XYZ-drives with Micrometer precision, individually Z-axes for print tools and optical components
- Decentralized units for printing, media control and computing save your precious space in biosafety cabinets and ensure superb heat dissipation
- Same software for all GeSiM bioprinters with easy to use CAD import
- UV-light sources of different intensity/ costs on the print head; camera systems
- Standard plastic cartridges as well as all Luer- lock nozzles can be used on all GeSiM bioprinters for 3d prints at room temperature
- Automatic alignment of all nozzle sizes to the building platform
- Print beds for SBS well plates with easy click fixation, temperature control and customized layouts available on request
GeSiM bioprinters are easy to operate in routine use:
Fully automatic adjustment of nozzles to the print bed (Left), the print bed size of BS5.1 (Right) fits to at least four SBS well plates or equivalent objects.
Nanolitre Pipetting
Tissue Engineering often raises interface and surface questions: How do cells adhere to artificial bones, implants and similar objects? What is the optimum scaffold geometry/ scaffold material to achieve appropriated cell adherence? Which cell line works, which doesn’t? Just throwing your ready-printed 3D object into a petri dish with cell suspension? – The Nanolitre pipetting module applies cells with spatial resolution during printing. Design and realization of complex artificial tissue with several cell types – partially applied to different parts of your 3D structure – becomes feasible.
The GeSiM Nanolitre Pipetting module allows up to 96 different cell suspensions or protein solutions for embedding into 3D structures during printing.
- CAD – based tethering of cells/proteins to parts of a 3D structure during printing,
- Volumes <100 Microlitres per species save resources when using rare and expensive samples,
- Onboard washing allows subsequent liquid handling of different species with one tool,
- Dispense volume ranges from 0.1 Nanolitre to Microlitres,
- Twin adapter for micro pipets enables parallel aspiration of two species with subsequent droplet mixing at the target position.
Refer to the tab PRINT TOOLS for technical information on available dispensers.
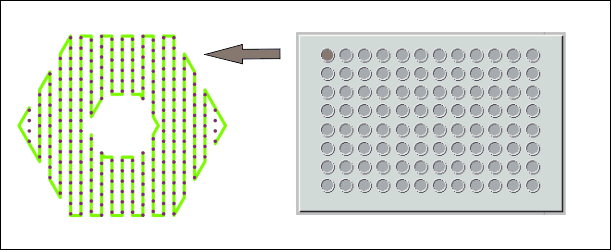
The pipetting module transfers up to 96 samples on 3D structures during printing
Sterile Working
Tissue Engineering assembles living cells to biopolymers. 3D printing shall be done quickly, safe and in a sterile environment. Physiological conditions have to be maintained to protect your particular cell lines which often incorporate a lot of labour and resources.
GeSiM Bioprinters always come without lid/ HEPA filters (Except BS5.3/CB). Our instruments are designed to be placed in a professional Biosafety Cabinet (BSC) or at least in a laminar flow hood. Many of these containments offer bench space for the manual preparation of bioinks immediately before 3D printing. It eliminates contamination risks when transferring your “living bioink” from another place to your 3D printer.
GeSiM collaborates with manufacturers of appropriated devices if you don’t have one in your lab. When space matters, think about the BS5.3/CB.
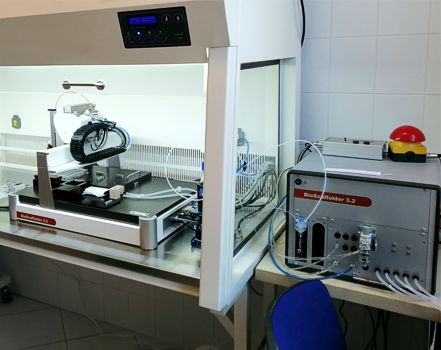
The BS3.2 bioprinter in a TELSTAR Aeolus laminar flow hood
GeSiM BioScaffold printers can be configured by numerous tools for different extrusion principles as well as optical components, like cameras and UV lamps.
The range of tools is continuously developing; please contact us for the latest catalogue. Bioinks and polymers with complex physical properties can be tested in advance for an optimum system configuration.
Always on board: Pneumatic Extruders
The BS3.3 Prime comes with two, all other models will have three pneumatic extruders on board. Pneumatic extrusion is a gentle method to 3Dprint strands of bioinks. All printing parameters can be set individually for each extruder. The pneumatic extruders accept 10mL plastic cartridges. Adapters for 1mL, 3mL, 5mL and 30mL are available.
Materials like Hydrogels and thermoplastics require temperature-controlled print heads. The following add-ons are optionally available for the 10ml cartridge extruder of each GeSiM bioprinter:
- Peltier cartridge chiller, 4°C to 80°C (left)
- Cartridge heater, RT to 190°C (middle)
- Nozzle heater (extends the cartridge heater), RT to 250°C (not shown)
Fast and precise heat transition is achieved by our proprietary stainless-steel cartridges, available in two sizes: 10mL and 1.5mL. Stainless-steel nozzles are available as well, with sizes from 0.1mm to 1.5mm.
Cartridge Stirrer/ Mixing Head
Cell suspensions may tend to sediment over time, particularly when large cells are suspended in low-viscous bioinks. The 10mL pneumatic extruder can be topped with a cartridge stirrer: A rotating spiral ensures a good mix-up of low-viscous bioinks (for operation at room temperature).
Mechanical Piston Extruders
Mechanical piston extruders offer volumetric displacement dispensing at constant flow rate, independent on material properties and cartridge size. They are beneficial for…
- Prints of very low-viscous bioinks,
- Prints of highly visco-elastic thermoplastic materials (E.g. PLA/bioglass compounds),
- All biopolymers performing insufficiently with the pneumatic printing system,
- Precise mixing of two components from different cartridges (short pot-life), with mixing ratio adjustable by software.
All GeSiM piston extruders offer sophisticated start/stop routines to compensate hysteresis of elastic biopolymers for exact strand deposition.
The High-Temperature Piston Extruder (HT-Extruder) prints thermoplastics like PLA (Polylactic Acid) and ABS at temperatures up to 250°C. It comes with a 10 Millilitre stainless-steel cartridge and stainless steel nozzles. A two-zone heater avoids the temperature drop from the cartridge to the nozzle. Nozzles with diameter ranging from 100 Micrometers to 1,500 Micrometers are available.
The 50mL Syringe Extruder is the less complex counterpart of the HT-Extruder. It operates at room temperature but takes disposable 50 Millilitre syringes for longer prints. Adapters for 30mL, 10mL and even smaller syringes are available.
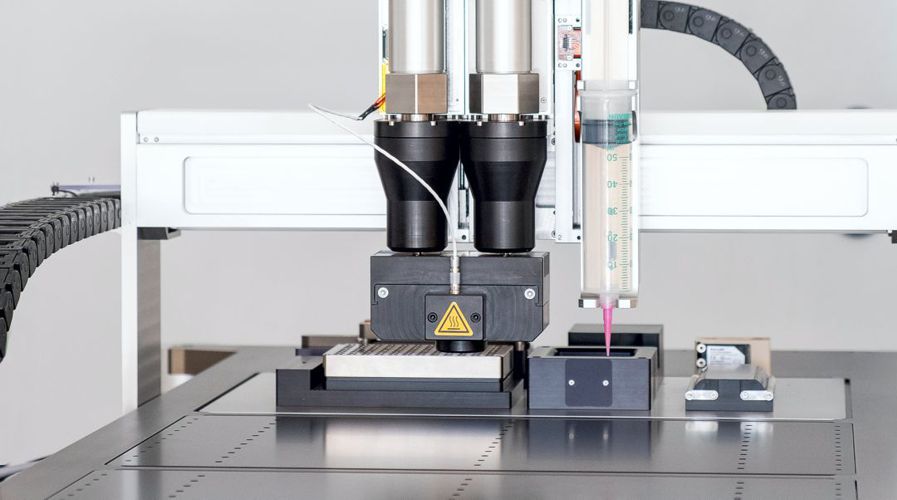
Piston Extruders on BS5.1: Pair of two HT-Extruders with gradient mixer (left); 50 mL Syringe Extruder (right)
A pair of GeSiM syringe extruders hooks up to mixing chambers when pre-print mixing of two materials in a single cartridge is not possible. The mixing chamber of the HT-Extruder heats up to 250°C, the mixing chamber of the 50mL syringe extruder operates at room temperature.
The BS5.x will be required to operate more than one HT-Extruder on the tool head.
PUREDYNE® Screw-Extruder
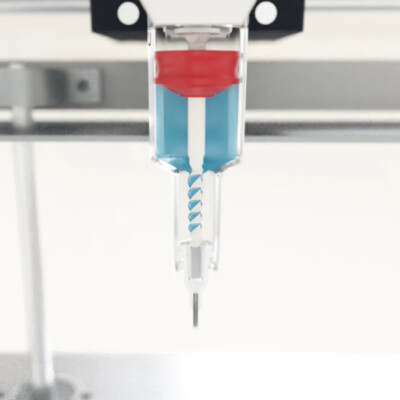
PUREDYNE Screw Extruder from ViscoTec GmbH (Click here for video)
Now it can be used with all GeSiM bioprinters. The Puredyne extruder masters small volumes of „challenging“ bioinks, difficult to be printed through our pneumatic extruders.
Core/Shell Extruder for coaxial printing of two materials
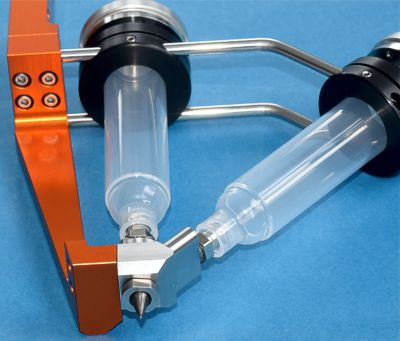
Core/Shell extruder for two materials
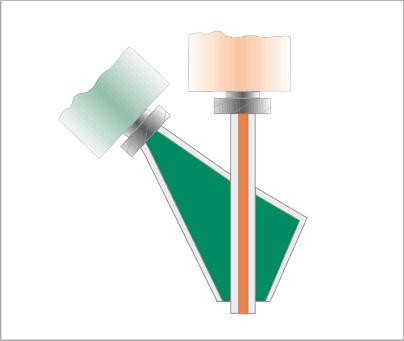
Schematic function
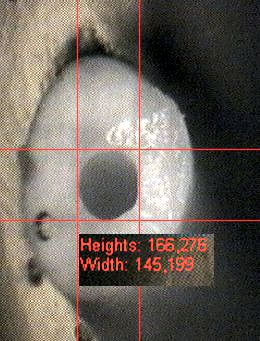
SEM image of C/S strand with empty core lane
Soft matrices like Hydrogels are cell friendly materials but hard to print into stable 3D structures. The combination with more stiff thermoplastic scaffolds may cause biocompatibility issues. The pneumatic C/S-extruder (Core/Shell-extruder) for the BioScaffold printers allows simultaneous printing of two materials with different properties in a coaxial arrangement. The tool features a pair of nozzles – an inner core nozzle, surrounded by the outer shell nozzle. The print parameters can be set individually for both channels in order to match properties of the respective bioinks. Nozzle diameters range from 100 Micrometre to 5000 Micrometre.
![Bioprinter 16 Core/Shell strands with cells inside after 7 days cultivation (Bright field image) [1]](https://gesim-bioinstruments-microfluidics.com/wp-content/uploads/2019/07/CS_Bright_Field_Image.jpg)
Core/Shell strands with cells inside after 7 days cultivation (Bright field image) [1]
![Bioprinter 17 Nuclei stained blue [1]](https://gesim-bioinstruments-microfluidics.com/wp-content/uploads/2019/07/CS_Stained_Cell_Nuclei.jpg)
Nuclei stained blue [1]
Centre for Translational Bone, Joint and Soft Tissue Research, Technische Universität Dresden
Nanolitre Pipetting Tools
The GeSiM bioprinters BS3.3 and BS5.x are available with the optional Nanolitre pipetting module. It transfers small liquid sample amounts from a 96 well micro titer plate to a defined locations on the target object during 3D printing.
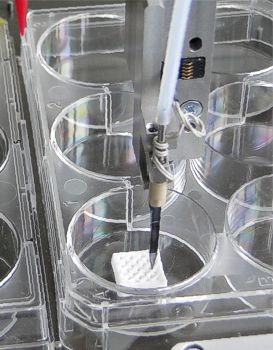
Piezo pipet on a 3D print
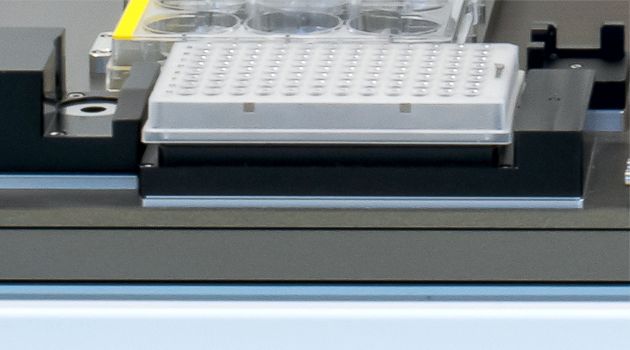
Source plater heater with 96 well plate; Drop camera (left)
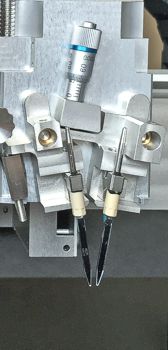
Pair of piezo pipets on a swivel (Twin tip)
Pipets for different volume range are available:
- Passive needles for syringe supported displacement dispensing. The lowest dispense volume is in the range of half a Microliter.
- The volume range between fifty Nanolitres and a few Microlitres is covered best by the solenoid dispense valve.
- Piezoelectric nozzles for free-flight micro drop dispensing (Viscosity limit around 10 mPa*s). The lowest dispense volume is in the range of 0.1 Nanolitres.
The innovative Twin-Tip-Pipettor operates two separate GeSiM piezoelectric nozzles on a swivel. For aspiration both nozzles dip into separate wells of a 96-well-plate. The droplets of both nozzles than hit each other and mix up on the target surface.
Heatable versions of the piezoelectric nozzles are available for liquids with higher but temperature dependent viscosity.
Curing/ Photopolymerization
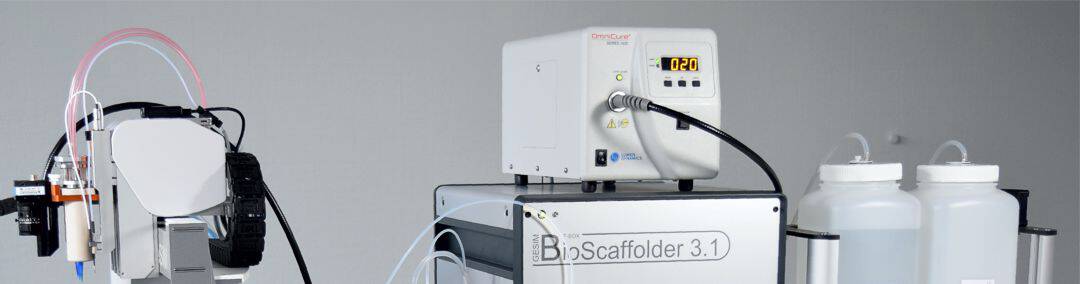
Omnicure S1500 lamp on BS3.1; Optical fibre on the print head
GeSiM sells the Omnicure S1500 UV lamp with the BioScaffolder instruments. An optical fibre connects the lamp main unit with the optics on the print head. The Omnicure S1500 comes with replacable band width filters. It is a very powerful UV lamp for quick hardening of UV-curable bioinks.
Single wavelengths UV-LED pens are the cost-attractive alternative. They cover the range from 265nm to 405nm. A “Blue Pen” (450nm) is available as well.
Melt Electro Spinning-Writing (MEW)
The optional MEW module for the BioScaffold printers BS3.2 and BS5.x combines pneumatic extrusion and high-voltage induced fibre deposition for particular thermoplastic materials. Strand size and layer thickness come down below 100 Micrometres. Strand thicknesses of 10…20 Micrometres can be achieved from materials like PCL with a molecular weight of up to 50,000.
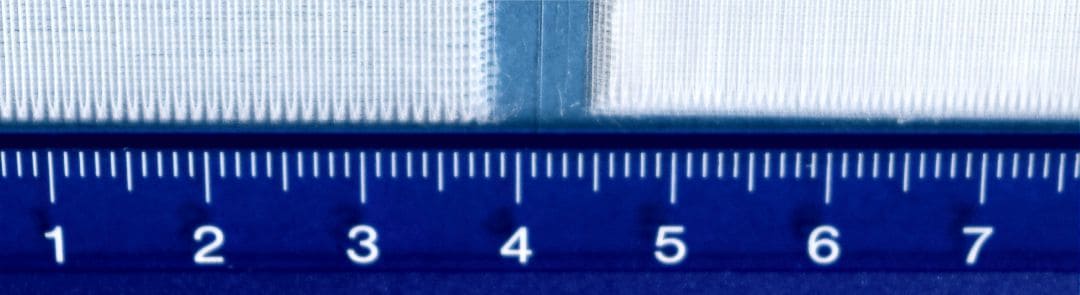
Micro-Mesh from PCL 50,000l 100 layers. The strand distance is 500 Microns (Left side) and 200 Microns (Right side)
In contradiction to Melt Electrospinning (MES) Melt Electrospinning Writing deposits each fibre in a regular manner. CAD geometries can be printed to a certain extent. GeSiM bioprinters combine MEW with pneumatic extrusion for a single print. E.g. grids at macro scale can be added to thin meshes made by MEW, achieving mechanical stiffness as well as good conditions for cell adhesion with the same 3D object.
The MEW module extends the standard configuration of the instrument by a special tray with embedded electrode, a high-voltage generator and security measures (left). Four different materials are print and spun to make one object (right).
Fused Deposition Modeling (FDM)
FDM belongs to the oldest methods for 3D printing. Instead of refillable cartridges filaments of defined diameter supply the print head in a continuous manner. On the other hand, available filaments are mainly made for mechanical engineering, with limited biocompatibility.
Optional available FDM print heads for the BioScaffold printers BS3.3 and BS5.1 extend the capabilities of our instruments beyond “pure bioprinting”.
Powder Pipet (EPO 3378574)
Tiny amounts of granular solid materials can be added to 3D prints by the new powder pipet: It’s kind of a vacuum tweezer with a defined pore chip or micro basket on the foot side. It aspirates either single grains or a few micrograms from a reservoir and dispenses it on a target. The foot area of the powder pipet is customized for a particular granule and application.
A particular biopolymer is usually available with very different compositions, molecular weight and physical properties (melting points, viscoelasticity). Please download the Tools at a Glance brochure for more information. GeSiM is ready for print tests with your own bioink/ biopolymer. Please contact us for arrangements.
Plasma Pen
The Nadir Plasma Pen (developed and manufactured by Nadir S.r.l.) is an atmospheric plasma jet that allows the ionization of Argon gas by applying high voltage (HV) near the channel where the gas is flowing. It can be configured to each GeSiM bioprinter as individual tool.
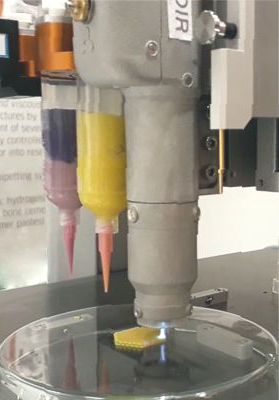
Plasma Pen on BS5.1
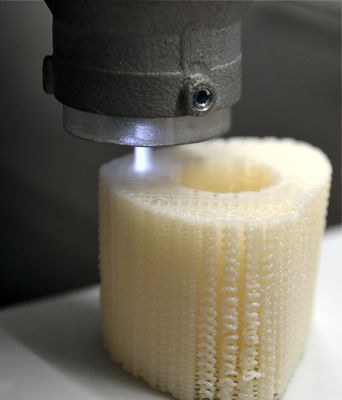
Plasma treatment of a bone biopolymer scaffold during printing
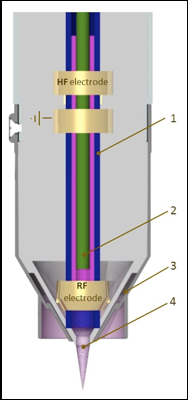
Schematic of Nadir Plasma Jet Module: Argon Channel (1), carrier gas or reactive gas capillary (2), cooling or shielding gas channel (3), plasma plume (4)
Applications:
- Activating and cleaning the whole object porosity
- Sterilization with oxidative plasma during polymer printing
- Functionalization with a proper chemical precursor without solvents
Benefits:
- Reductive or oxidative plasma can be used to clean surfaces, remove organic dirt or polymeric layers
- Surfaces can be activated to improve adhesion of cells, printed material or different melted materials
- Inlet capillary for the introduction of vapour and aerosol precursors allows deposition of functionalities, coatings and nanocomposites
The Nadir Plasma Pen was developed and adapted to GeSiM products within the frame of the European FAST project. Please download the FAST brochure for more information.
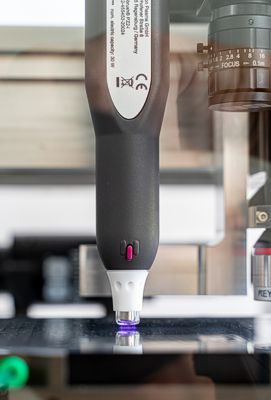
Plasma Pen made by Relyon
The Relyon Plasma Pen is the cost-effective alternative with limited functionality: It activates/cleans surfaces, without the capability of depositing reagents from ionized gas.
Please download the BioScaffolder brochure for more details on available tools or just contact us for full catalogue with all technical details and specs.
Highest Flexibility for a Changing World
GeSiM instruments incorporate highest modularity. Even when acquired for a particular project, additonal tools for upcoming projects are available at affordable costs.
Our budget line BS3.3 Prime comes with a fix combination of tools, whereas BS3.3 and BS5.3 can be configured almost arbitrarily. Here we show popular setups, please visit the preceding tab “Print Tools” for more information or download the brochure below.
Hardware upgrades mostly can be done without a service visit of GeSiM engineers (Limited for BS3.3 Prime). GeSiM permanently developes new tools/modules, probably fitting to your personal BioScaffold printer. The user software GeSiM Robotics reconfigures easily and reflects always the actual status when adding new hardware. Tool-related pieces of software can be added similar to the download of apps for your phone.
The GeSiM factory service is available for older BioScaffold printers as well as for retrofitting of BS3.3 Prime with fix configuration.
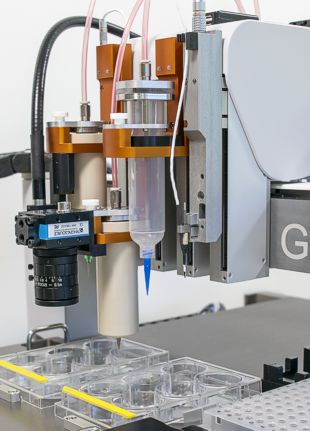
2x 10mL cartridge with heaters; Camera; 30 mL cartridge, Piezo Pipet (From left)
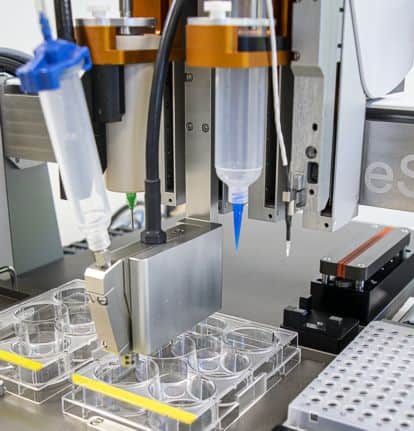
10mL cartridge with heater and UV head; Piezo valve (High viscosity); 30mL cartridge; Piezo Pipet (From Left)
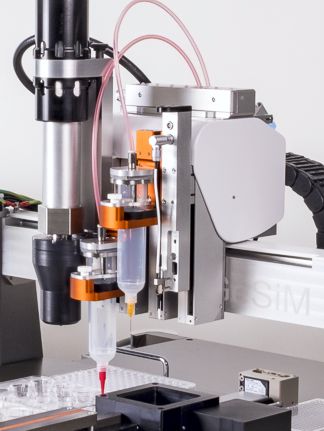
HT-Extruder; 2x 30mL cartridges; Piezo Pipet (From left)
Particular applications may require tools for high pressure, high temperature, extremely thin strands and others:
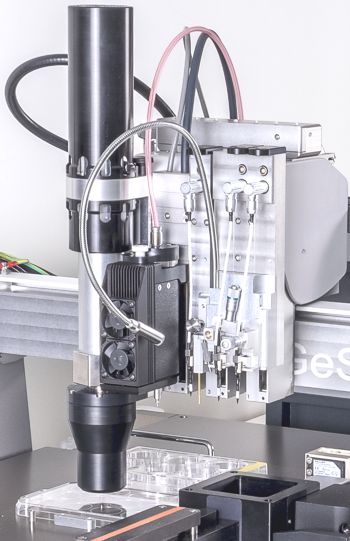
HT-Extruder; 10mL Peltier chiller; Z-sensor; Twin Piezopipet (From left)
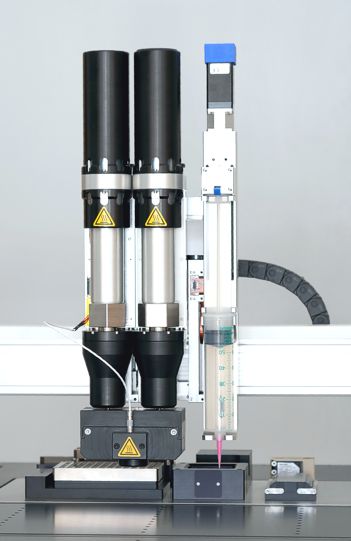
HT-Extruder 2x with gradient mixer; 50mL Extruder (From left)
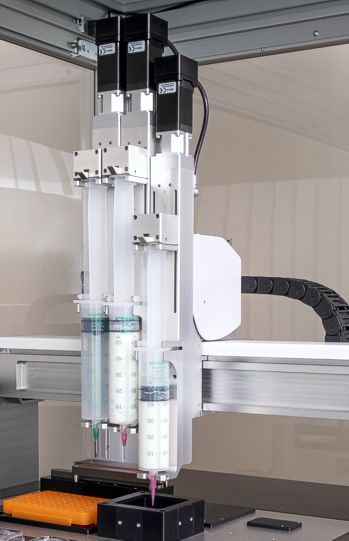
50mL Extruder 3x, for printing of large models and batch production
The Multi-Material Printer: (1) Two syringe extruders with mixing head, (2) FDM print head, (3) Droplet dispenser/ UV-pen, (4) Multi-tool axis with Keyence layer tickness measurement, camera, plasma pen. Additional tools arranged on the work plate: (5) Stroboscopic camera for drop inspection, (6) Levelling table with substrate fixation. A sophisticated version in a closed cabinet with HEPA-filter; suited for applications in the field of polymer electronics
GeSiM Robotics
The user software of all our Bioprinters is called GeSiM Robotics. It is a flexible but intuitive software:
- Configuration and automatic alignment of print tools and print targets
- Quick test prints considering varying print parameters give best settings for a particular combination of cartridges, nozzles and biopolymers
- Optical monitoring of drop-on-demand pipets
- Replacement of cartridges/ nozzles on demand during a 3D print eliminates the risk of unsuccessful runs due to clogged nozzles and run out cartridges
- Planning and execution of batch processes (sequences) allows copies of a 3D structure or different separate 3D structures unattendedly.
Processing of External CAD Data
GeSiM Robotics supports STL and 3mf formats. An STL file describes triangulated surfaces in a three-dimensional Cartesian coordinate system. It is widely used for rapid prototyping, 3D printing and computer aided manufacturing.
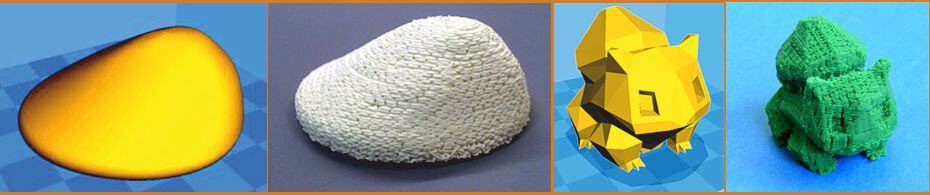
3D models printed from STL files: Women breast model (left), bulbasaur (right)
The BioScaffold printer software defines internal scaffold- structures in between surfaces imported from a STL file. The 3mf format works similar but supports different objects in one file. It is the preferred data standard for multi-material scaffolds.
Printing Life to Shape the Future
Beside of instrumentation, 3D printable materials are the next key to success in tissue engineering. Over the past decade research teams worldwide focused on tuning quite common materials like gelatine into 3D printable bioinks. Opposing specs like cell friendliness and mechanical stiffness have to combine for one product.
GeSiM bioprinters accompanies several of these groups, therefore contributing to the scientific progress. Like most bioprinters, GeSiM BioScaffold printers accept all commercially available bioinks, granules, powders, pastes, liquids and filaments.
In order to offer all components for tissue engineering from one source we collaborate with InnoRegen, Inc., based in Daegu, Korea. GeSiM is selling InnoRegen Products and will be happy to discuss your particular application. Please contact us for price quotation on InnoRegen Bioinks.
InnoRegen Hydrogels
Prints best at temperature between 4°C and room temperature, e.g. with the GeSiM Peltier module.
Gel4Cell
Bioink for 3D bioprinting with gelatine hydrogel for increased biocompatibility and structural safety applied throughout the study of regenerative medicine.
- Cell compatible material
- High printability and uniformity
- UV-cross linkable for structural stability
Gel4Cell – Peptides
Peptide conjugated hydrogels supporting cell growth with particular tissue types.
- Gel4Cell – BMP: Bioink, which can be applied to bone regeneration research by combining BMP-2 peptide, a bone regeneration growth factor.
- Gel4Cell – VEGF: Bioink, which can be applied to vessel and skin regeneration research by combining VEGF peptide, an angiogenesis factor.
- Gel4Cell – TGF: Bioink, which can be applied to cartilage regeneration research by combining the cartilage regeneration growth factor TGF-β1 peptide.
Gel4Tissue
Bioink, which can be applied to artificial organ/tissue regeneration studies by combining pig’s intestinal membrane extracellular vagina (SIS-ECM).
Col4Cell
Collagen hydrogel for increased biocompatibility and structural safety.
InnoRegen Thermoplastic Biopolymers
Meltable biopolymers with good fit to the GeSiM cartridge heater and HT-Extruder.
- Polyinks – PCL: Caprolactone Synthetic Polymer with excellent biodegradability. For use with cells Gel4Cell bioink can be used within the same print. The printing temperature is between 65°C and 100°C.
- Polyinks – PLA: L-lactide synthetic polymer with excellent biodegradability, printing temperature is between 200°C and 250°C.
- Polyinks – PLCL: A blend of PCL and PLA with controlled biodegradability, printing temperature between 70°C and 250°C.
Biodegradability:
PLCL(W): 2….6 weeks PLCL(M): 6…10 weeks PLCL(Y): 10…16 weeks
Content
- Introduction
- Melt Deposition Modelling of Bioactive Organic/Inorganic Hybrid Scaffolds with HT-Extruder
- MEW Milk Protein/PCL Blends for Skin Regeneration
- Melt Electro Writing Scaffolds seeded with Stem Cells
- PCL-PEG Blends for Tissue Engineering
- Struts and Capsules
- MEW spun PCL Structures Mimic Artificial Eardrums
- Green Bioprinting
- Alginate/Methylcellulose Blends
- Bioprinting of Functional Islets of Langerhans
- Bioprinting: The Future of Surgery?
- As FAST as Possible
What are the GeSiM BioScaffold printers used for?
– Production of support structures (Scaffolds…) for tissue engineering – Printing of 3D bodies from biopolymers – Research and development of biopolymers for sustainability and environmental protection |
– Application of living cells and bacteria, either embedded in a biopolymer or seeded by the optional pipetting unit – 3D Printing of ceramic pastes and bone replacement materials – Melt electro spinning writing for research on lymph nodes and eardrums, |
This section presents highlights of the work of our customers with GeSiM instruments for bioprinting. It is neither comprehensive nor can GeSiM be responsible for content and correctness.
Melt Deposition Modelling of Bioactive Organic/Inorganic Hybrid Scaffolds with HT-Extruder
Research at the Laboratoire de Physique de Clermont, France is focused on the development of biomaterials with tailored bioactive, osteostimulative and other therapeutic properties for bone repair and regeneration. In particular, key studies focus on the design of an organic/inorganic platform technology inspired by the complex physiological structure of the bone: hybrids materials combining polymers and inorganic species at the molecular scale. The components of such materials can be modified to obtain a wide range of formulations with diverse physicochemical and biological properties. This, in turn, highly influences the processing conditions of each hybrid formulation into its final form.
Investigations are being carried out to understand and tailor the processing into purposefully designed 3D implantable scaffolds with microporosity and nanotopographical features that can aid cell adhesion and in-growth of tissues and vessels. After successful results using conventional scaffold fabrication techniques (Bossard et al., 2018, Biomedical Glasses), our current studies focus on the possibility to apply additive manufacturing (AM) to organic/inorganic hybrid. The optimization of AM for hybrids can open up to new shapes and structures, helping to achieve topographical features, and possibly clinical applications, that are currently unattainable with other techniques.
The Bioscaffolder 3.2 by GeSiM proved itself to be an adequate platform for our purpose. Thanks to its modularity, it is easy to quickly perform the testing of several candidate materials with different set-ups, often in parallel. It is possible to run proof-of-concept small batch trials for several newly synthesized formulations, optimizing time and resources. In particular, current results, although at their early stages, confirmed that with careful setting of the HT extruder module it is possible to obtain thrust forces that are sufficient for the extrusion of bioactive hybrids of polycaprolactone and bioactive glass, through both 500 and 400 μm nozzles. The temperatures and flow rates used ranged between 80-120 °C and 3-5 μm/s, respectively, depending on the molecular weight of the polymer used. With these parameters the successful direct printing of a bioactive glass hybrid was performed for the first time.
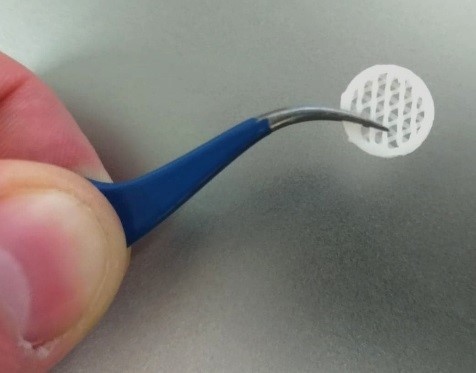
PCL/ Bioglass scaffold in a tweezer
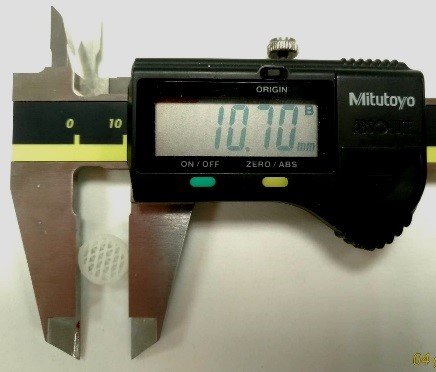
The test object at 10mm size
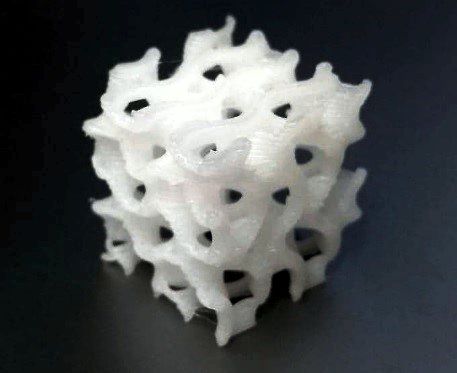
Internal porous structure of a hybrid scaffold
Courtesy of: Dr. Lukas Gritsch, Prof. Jonathan Lao
Universite Clermont Auvergne,
Laboratoire de Physique de Clermont,
CNRS/IN2P3, Biomaterials group,
France
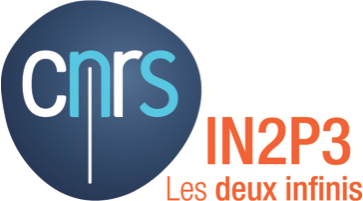
References:
[1] H. Granel, C. Bossard,, J Lao, Y. Wittrant, et al – Bioactive Glass/Polycaprolactone Hybrid with a Dual Cortical/Trabecular Structure for Bone Regeneration – ACS App. Biomater. 2(8), 3473-3483, 2019. [2] Cédric Bossard, Henri Granel, Édouard Jallot, Christophe Vial, Hanna Tiainen, Yohann Wittrant, J. Lao – Polycaprolactone / bioactive glass hybrid scaffolds for bone regeneration, Biomedical Glasses 4(1), 108-122, 2018.MEW Milk Protein/PCL Blends for Skin Regeneration
The GeSiM bioprinter BS3.1 is an important tool at the Center for Bioengineering and NanoMedicine at the University of Otago, New Zealand.
Demand for skin replacements is rapidly increasing as burn and full-thickness wounds are difficult to repair due to the low regeneration capability of innate tissues, as well as the physical drawbacks associated with currently available substitutes.
Polycaprolactone (PCL), a bioresorbable and biocompatible, synthetic polymer was selected as scaffold material due to its mechanical stability, flexibility, and superior melt processing properties. In order to increase PCL’s biological functionality bioactive milk proteins (MPs) were blended with PCL. To date (August 2019, GeSiM), this is the first study of its kind detailing the tissue regenerative capacity of PCL containing MPs as bioactive additives for skin regeneration using MEW. The aim of this study was to MEW MP/PCL tissue engineered constructs (TEC) and assess their suitability for generating tissue in vitro.
The MPs, lactoferrin (LF) and whey protein (WP), were mixed with PCL individually at varying concentrations (0.05%, 0.1%, 0.25%), and in combination (COMB) at concentrations of 0.25% e ach. TECs were characterized chemically, physically, and their biological activity assessed in vitro. Physical characterisation of MEW MP/PCL scaffolds showed that reproducible, layered micron range scaffolds could be fabricated; displaying high porosity, low degradation, and rapid protein release. Biological activity, determined via an in vitro skin model using human keratinocytes (HaCaTs) and normal human dermal fibroblasts (Nhdf) cells, showed significantly increased cell growth, spreading, and infiltration into LF (0.25%) containing scaffolds and COMB scaffolds when compared to PCL alone (p≤0.05). These findings demonstrated that the combined addition of LF and WP increased the biological activity of MEW PCL scaffolds and could be potentially used as a TEC for deep tissue dermal regeneration.
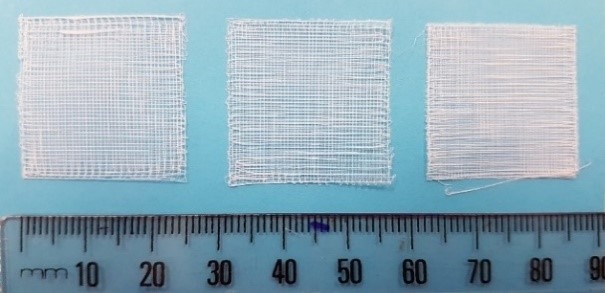
Visual comparison of scaffolds. Scaffolds generated as a result of setting pore size parameters on the GeSiM BioScaffolder 3.1 software. From left, the pore size parameters were set to 500 x 500µm, 400 x 400µm, and 300 x 300µm.
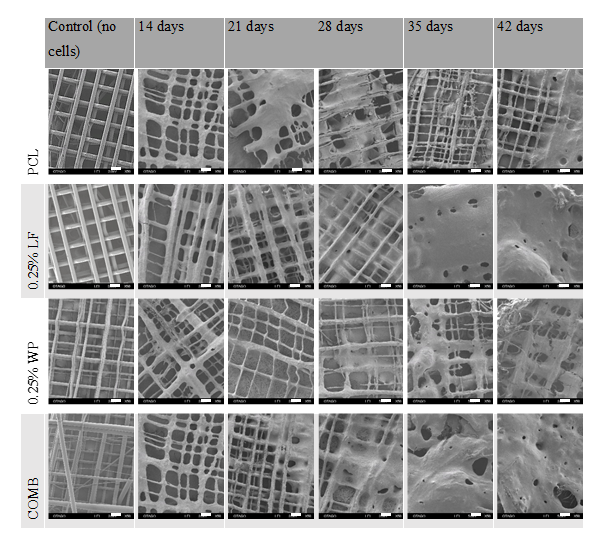
SEM micrographs of scaffolds cultured at 14, 21, 28, 35, and 42 days. Scale bar = 200 µm
Melt Electro Writing Scaffolds seeded with Stem Cells
Melt Electro Writing (MEW) allows the creation of micron level pore sizes as compared to syringe powered pneumatic extrusion. Cells preferentially prefer these smaller pores as shown in this study where thin 4 layered MEW PCL (50kDa) test scaffolds of varying pore size (250 or 450 µm) were made.
Preparation and processing of scaffold tests using the sandwich MEW technique:
(A) Scaffold cMEW sandwiches are made by placing a cell-laden cMEW between two sheets of test scaffold (17.5 · 17.5 mm) and spot welding them together as indicated, using a flame-heated metal rod. cMEW sandwiches were then allowed to grow in BSA-coated Petri
dishes for 3 weeks.
(B) cMEW sandwiches were processed as shown after incubation. Separation and trimming are performed before fixation with TCA, weighing, and SRB staining.
(C) cMEW sandwiches were visualized and photographed live under bright field microscopy at various time points to track cell area and maximum distance travelled. Images were stitched together for analysis as given in Figure 4.
(D) A single batch print of nine cMEW templates fabricated before trimming with scissors. Bar = 5 mm. SRB, sulforhodamine B; TCA, trichloroacetic acid.
The authors developed a special method of monitoring mesenchymal stem cell (T0523) proliferation and migration by seeding cells on circular MEW (cMEW) discs as defined starting points sandwiched between two opaque PCL test scaffolds. The smaller pore size test scaffolds exhibited faster cell growth, spread, and expansion. This novel MEW sandwich technique could potentially be used to quantitate cell numbers and migration in other 3D multi-layered MEW scaffold systems.
Seeding cMEW scaffolds:
(A) Polystyrene culture vessels were either tissue culture treated or untreated and were coated or not coated with 0.1% BSA in PBS overnight before addition of T0523 cell, arrow points to 90 mm spheroid.
(B) Spheroid viability (i) T0523 spheroids transferred from A (iii) to tissue culture-treated flask; (ii) T0523 cells from A (iv) to tissue culture-treated flask.
(C) cMEW fixed and stained with SRB. Cells can be seen coating scaffold and filling pores.
(D) Fluorescent image of T0523 cells attached to cMEW scaffold. Green = GFP, Red = phalloidin conjugated to Alexa Fluor 594, Blue = Hoechst 33342. (A–C) Scale bar = 250 mm. (D) Scale bar = 100 mm.
References:
[1] Paul R. Turner, Minami Yoshida, M. Azam Ali and Jaydee D. Cabral: Melt Electrowritten Sandwich Scaffold Technique Using Sulforhodamine B to Monitor Stem Cell Behavior, TISSUE ENGINEERING: Part C, Volume 26, Number 10, 2020A/P Azam Ali, Director – Bioengineering Programme & Centre for Bioengineering & Nanomedicine (Dunedin Hub), University of Otago, Dunedin, NZ
Dr. Jaydee Cabral, Senior Lecturer, Centre for Bioengineering & Nanomedicine (Dunedin Hub), University of Otago, Dunedin, NZ
PCL-PEG Blends for Tissue Engineering
PCL (PolyCaproLactone) is a popular hard-phase biopolymer for tissue engineering and 3D printing. It is biocompatible and – to a certain extent – biodegradable. Multi-printhead instruments like the GeSiM BS31 easily combine PCL struts with cell friendly alginate/hydrogels.
Hard-phase biopolymers shall be optimized towards a quick degradation/mass loss when getting in contact with body fluid. An inherent drawback of pure PCL is the relatively high stability under physiological conditions. Here we present a recent study [1] addressing this problem. It was conducted using the predecessor of BS3.1, BS2.1.
PCL and polyethylene glycol (PEG) blends (PCL-PEG) together with alginate dialdehyde gelatine hydrogel (ADA-GEL) loaded with stromal cell line (ST2) were investigated.
![Bioprinter 45 Scheme of a hard-soft phase scaffold with the hard thermoplastic phase (grey) and the soft hydrogel phase (yellow) containing the cells [1]](https://gesim-bioinstruments-microfluidics.com/wp-content/uploads/2019/07/Two-materials-scaffold-design.png)
Scheme of a hard-soft phase scaffold with the hard thermoplastic phase (grey) and the soft hydrogel phase (yellow) containing the cells [1]
![Bioprinter 46 Stereomicroscope images of a plotted PCL-PEG (7030) scaffold as fabricated: topview (a); and side view (b) (scale bar = 2 mm) [1]](https://gesim-bioinstruments-microfluidics.com/wp-content/uploads/2019/07/Hard-phase-scaffold.png)
Stereomicroscope images of a plotted PCL-PEG (7030) scaffold as fabricated: topview (a); and side view (b) (scale bar = 2 mm) [1]
![Bioprinter 47 Fluorescence microscope images (a–f) of the actin cytoskeleton (red) and the cell nuclei (green) of ST2 cells in a PCL-PEG ADA-GEL construct after 28 days of incubation of different magnification: (a,b) overview images; (c) densely packed area of the cells covering both materials; (d) cell morphology on the hard phase; (e) cell agglomerate and spread single cells in hydrogel; and (f) densely packed area of cells (hydrogel phase) [1]](https://gesim-bioinstruments-microfluidics.com/wp-content/uploads/2019/07/Fluorescence-image-cytoskeleton-nuklei.png)
Fluorescence microscope images (a–f) of the actin cytoskeleton (red) and the cell nuclei (green) of ST2 cells in a PCL-PEG ADA-GEL construct after 28 days of incubation of different magnification: (a,b) overview images; (c) densely packed area of the cells covering both materials; (d) cell morphology on the hard phase; (e) cell agglomerate and spread single cells in hydrogel; and (f) densely packed area of cells (hydrogel phase) [1]
References:
3D-printing of Cell-loaded Alginate Capsules suspended in Hydrogel
Printable biomaterials can benefit from complex compositions: The release of drugs or cell growth have to be controlled after printing. A group at the Friedrich-Alexander University in Erlangen added prefabricated capsules enriched with cells to hydrogel before printing.
The GeSiM BioScaffolder was part of this study. It presents a novel method to produce macroporous hydrogel scaffolds in combination with cell-loaded capsule-containing struts by 3D bioplotting.
This approach enables loading of the capsules and strut phases with different cells and/or bioactive substances and hence makes compartmentalization within a scaffold possible.
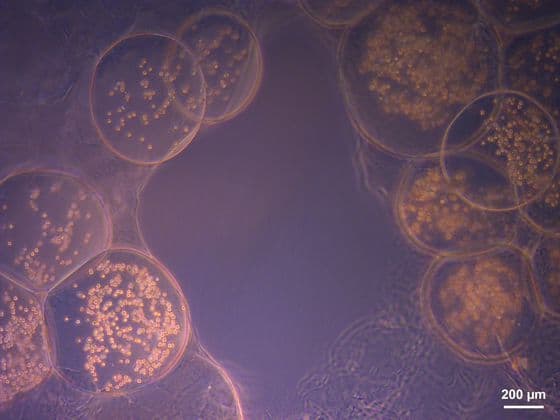
Light microscopy images of cell-loaded alginate capsules in ALP-loaded alginate struts immediately after fabrication. The free
space in the center of the image is a macropore.
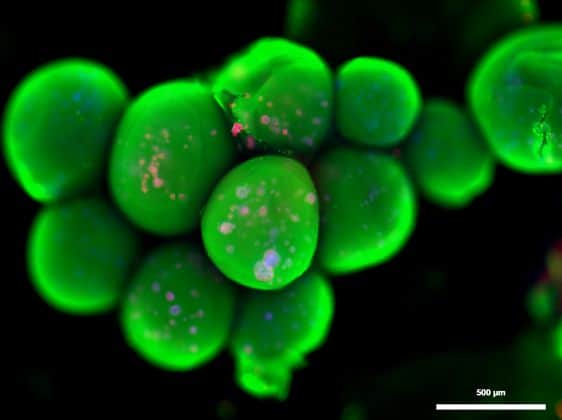
Fluorescence microscopy image after 10 days of culture and OsteoImage®, DAPI and Vybrant staining capsules loaded with ALP. Green: calcium phosphate. Blue: cell nuclei. Red: cell body. Scale bars: a = 200 µm, b = 500 µm
The goal was to produce scaffolds for possible applications in bone tissue engineering consisting of alginate struts containing alginate capsules enriched with MG-63 osteoblast-like cells and ALP (alkaline phosphatase). Two combinations were compared, namely ALP in the struts and cells in the capsules and vice-versa. Both combinations were cytocompatible for cells and mineralization of scaffolds could be detected in both cases, according to an OsteoImage staining. ALP had no adverse effect on cytocompatibility and enhanced mitochondrial activity.
Different components desirable for bone regeneration, e.g., cells and bioactive proteins, can be incorporated both in the capsules and struts. This enables compartmentalization of components, which facilitates greater flexibility in modification of the scaffold.
References:
Rainer Detsch, Bapi Sarker, Tobias Zehnder, Aldo R. Boccaccini and Timothy E.L. Douglas:
Additive manufacturing of cell-loaded alginate enriched with alkaline phosphatase for bone tissue engineering application. De Gruyter, BioNanoMat 2014; 15(3-4): 79–87
MEW spun PCL Structures Mimic Artificial Eardrums
Using the GeSiM BioScaffolder 3.1, the Melt Electrowriting (MEW) process (Figure 1) was used to produce thin scaffolds of various structures within the dimensions of the human tympanic membrane (TM). It could be shown that these scaffolds meet the necessary criteria for a possible TM replacement. For this purpose, medical polycaprolactone (PCL) was melted and processed to thin fibers with 10-15 µm in diameter.
The fiber deposition was realized in different layer number, fiber distances and layer-to-layer orientations (Figure 2A-B). The different designs were characterized based on their mechanical stability and vibration characteristics. Comparisons with the human tympanic membrane showed that depending on the design, the scaffold properties could mimic the TM properties.
The immanent pores of the MEW scaffolds were closed using a thin collagen layer (thickness~300-400 nm), which increased the transmission of lower frequencies but also the growth rate of human immortalized keratinocytes (HaCaT). The results recently have been published (von Witzleben et al., 2021).
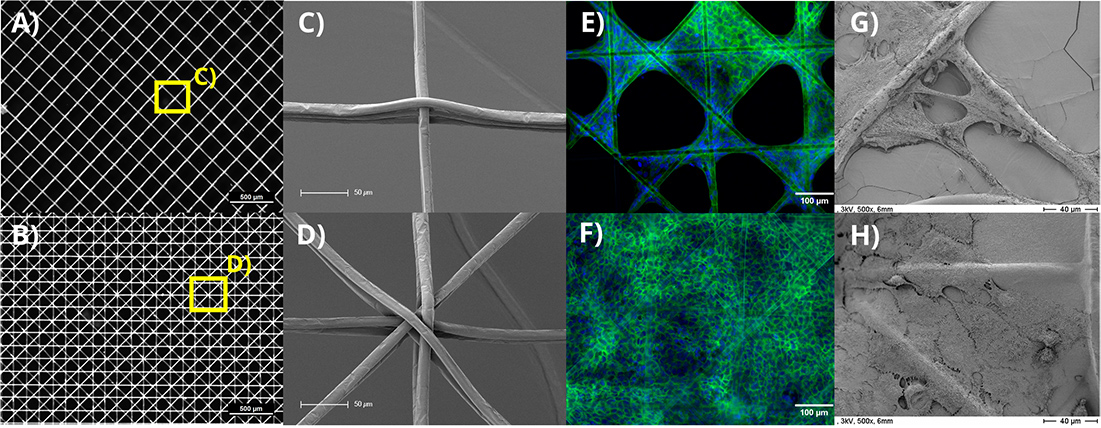
Figure 2: Examples of the different investigated designs with fiber diameters of 10 µm, 250 µm fiber distances. In A) 6 layers with a 90° layer-to-layer orientation and in B) 8 layers with a 45° layer-to-layer orientation are shown. Images C) and D) depict scanning electron microscopy images of the highlighted (yellow) intersections of A) and B), respectively. The growth of human keratinocytes (HaCaT) is shown in E) on blank MEW scaffolds and in F) on collagen coated scaffolds. The cell nuclei were stained blue (DAPI) and the cell actin cytoskeleton green (Phalloidin). SEM images of the identical scaffold types with HaCaTs are shown in the respective images labelled G) & H).
References:
M. von Witzleben, T. Stoppe, T. Ahlfeld, A. Bernhardt, M.-L. Polk, M. Bornitz, M. Neudert, M. Gelinsky: Biomimetic tympanic membrane replacement made by melt electrowriting. Adv. Healthcare Mater. 2021 (in press, DOI: 10.1002/adhm.202002089)
Fabrication of Photosynthetic Algae-laden Hydrogel Scaffold
The “Green Bioprinting” approach is expected to bring an advantage for existing applications of microalgae in the biotechnological field as, e.g. harvesting and separation procedures could be simplified and the co-immobilization of microalgae with (e.g. plant growth promoting) bacteria could be conducted in a spatially organized manner. In addition, this novel approach opens further possibilities for new, future-oriented applications such as the usage of microalgae or other plant cells in the medical field. The cocultivation of algae in close vicinity to human cells could enable a sustained delivery of oxygen or secondary metabolites with therapeutic potential to human cells without the need of external supply. The fabrication of patterned coculture scaffolds can be easily realized by two-channel plotting. [1]
In this study, conducted by GeSiM customers at the Centre for Translational Bone, Joint and Soft Tissue Research at the Technische Universität Dresden in collaboration with partners from the Institute of Bioprocess Engineering at the TU Dresden, a simple geometry was chosen to demonstrate embedding of microalgae in an alginate hydrogel scaffold by 3D plotting.
![Bioprinter 51 Algae loaden scaffold after 1 day of culture [1]](https://gesim-bioinstruments-microfluidics.com/wp-content/uploads/2019/07/Algen_Fig1A_day1.jpg)
Algae loaden scaffold after 1 day of culture [1]
![Bioprinter 52 Algae loaden scaffold after 12 days of culture [1]](https://gesim-bioinstruments-microfluidics.com/wp-content/uploads/2019/07/Algen_Fig1A_day12.jpg)
Algae loaden scaffold after 12 days of culture [1]
References:
1] A. Lode, F. Krujatz, S. Brüggemeier, M. Quade, K. Schütz, S. Knaack, J. Weber, T. Bley, M. Gelinsky: “Green bioprinting: Fabrication of photosynthetic algae-laden hydrogel scaffolds for biotechnological and medical applications”, Engineering in Life Sciences, Volume 15, Issue 2, pages 177–183, March 2015
[2] F. Krujatz, A. Lode, S. Brüggemeier, K. Schütz, J. Kramer, T. Bley, M. Gelinsky, J. Weber: „Green Bioprinting: Viability and growth analysis of microalgae immobilized in 3D-plotted hydrogels versus suspension cultures“, Engineering in Life Sciences, Volume 15, Issue 7, pages 678–688, October 2015Alginate/Methylcellulose Blends for 3D printing (Alg/MC)
The group of Prof. Michael Gelinsky at the Technische Universität Dresden conducted a study to overcome the limitations of biofabrication with cell-friendly TE Materials. The aim of the study was to develop a plotting material that is based on alginate, the probably most popular substrate material for biological 3D printing. The goal was to find a composition optimized both for printing and for cell embedding.
Basically a rather easy approach was used: Addition of Methylcellulose (MC) to low concentrated alginate. That leads to an enhanced viscosity and therefore improved printing conditions. The MC did not contribute to the gelation and was released from the scaffolds during the following cultivation. Mesenchymal stem cells were added to the alginate-MC blend and showed high viability after several weeks of cultivation within the plotted scaffolds.
![Bioprinter 53 3D plotting of alginate-based hydrogel scaffolds: (A) 3 wt% alginate without methylcellulose, four layers; (B) 3 wt% alginate + 9 wt% methylcellulose (alg/MC), four layers; (C) alg/MC, 20 layers; (D) alg/MC, 50 layers; (insert) top view. [1]](https://gesim-bioinstruments-microfluidics.com/wp-content/uploads/2019/07/AG-MC_Scaffold.jpg)
3D plotting of alginate-based hydrogel scaffolds: (A) 3 wt% alginate without methylcellulose, four layers; (B) 3 wt% alginate + 9 wt% methylcellulose (alg/MC), four layers; (C) alg/MC, 20 layers; (D) alg/MC, 50 layers; (insert) top view. [1]
References:
[1] Kathleen Schütz, Anna-Maria Placht, Birgit Paul, Sophie Brüggemeier, Michael Gelinsky and Anja Lode: Three-dimensional plotting of a cell-laden alginate/methylcellulose blend: towards biofabrication of tissue engineering constructs with clinically relevant dimensions, Journal of Tissue Engineering and Regenerative Medicine, Article first published online: 22 JUL 2015An Application for Alg/MC: Bioprinting of Functional Islets of Langerhans
The aim of healing Diabetes mellitus Type 1 by transplantation of pancreatic islets requires the encapsulation of said islets in semi-permeable materials for immune-protection. To achieve this with a single device in clinically relevant size, a macroporous construct consisting of a semi-permeable material with embedded islets would be beneficial.
In a joint research project with the Medical Clinic 3 of the University Hospital Carl Gustav Carus in Dresden, the Centre for Translational Bone, Joint and Soft Tissue Research conducted a study on the possibility of 3D printing scaffolds containing functional pancreatic islets. It was possible to demonstrate not only a high rate of viability within printed scaffolds, but for the first time also show functionality of bioprinted islets (Duin et al., 2019). The material this was achieved with was the slightly adapted Alginate/Methylcellulose blend previously developed by this group (Schütz et al., 2017). To achieve functionality of islets a high permeability of the material for glucose and insulin is required, which was likely improved by the microporosity the methylcellulose adds to the scaffolds (Hodder et al., 2019).
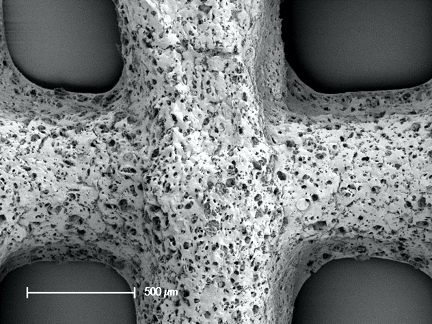
A) SEM image of an Alg/MC-scaffold depicting microporosity. Scale bar = 500 µm
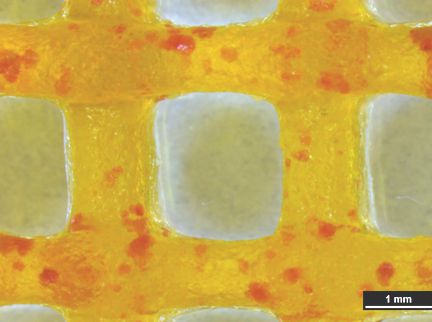
B) Light microscopic image of an Alg/MC-scaffold with porcine pancreatic islets stained for insulin
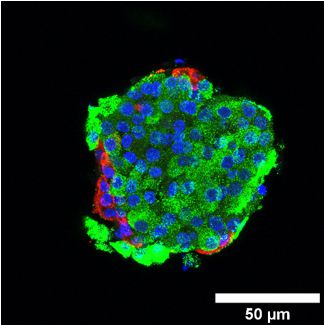
C) Fluorescence microscopic image of a pancreatic islet in a plotted Alg/MC-scaffold.
References:
[1] Duin, S., Schütz, K., Ahlfeld, T., Lehmann, S., Lode, A., Ludwig, B., & Gelinsky, M. (2019). 3D Bioprinting of Functional Islets of Langerhans in an Alginate/Methylcellulose Hydrogel Blend. Advanced Healthcare Materials, 8(7), 1801631. https://doi.org/10.1002/adhm.201801631 [2] Hodder, E., Duin, S., Kilian, D., Ahlfeld, T., Seidel, J., Nachtigall, C., Bush, P., Covill, D., Gelinsky, M., & Lode, A. (2019). Investigating the effect of sterilisation methods on the physical properties and cytocompatibility of methyl cellulose used in combination with alginate for 3D-bioplotting of chondrocytes. Journal of Materials Science: Materials in Medicine, 30(1). https://doi.org/10.1007/s10856-018-6211-9 [3] Schütz, K., Placht, A. M., Paul, B., Brüggemeier, S., Gelinsky, M., & Lode, A. (2017). Three-dimensional plotting of a cell-laden alginate/methylcellulose blend: towards biofabrication of tissue engineering constructs with clinically relevant dimensions. Journal of Tissue Engineering and Regenerative Medicine, 11(5), 1574–1587. https://doi.org/10.1002/term.2058Reconstruction of a Human Scaphoid Bone
The replacement of bones in course of accident treatment usually requires titanium implants. It is a widely used material for trauma surgery but shows inherent drawbacks: A mismatch of mechanical properties, interface issues to the surrounding soft tissues and no capability to grow.
A joint research project of GeSiM and Dresden University of Technology (TU Dresden) – Centre for Translational Bone, Joint and Soft Tissue Research – aimed in establishing 3D printing of patient-specific implants of a degradable biomaterial. As model the human scaphoid bone was selected and 3D data extracted from a CT scan.
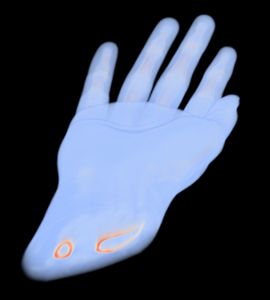
1a) CT data set of human hand
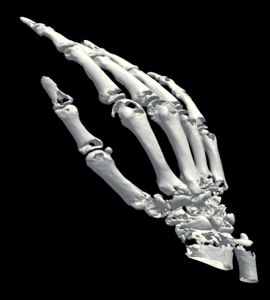
1b) Bone extraction
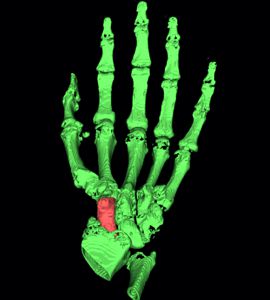
1c) Segmentation of the scaphoid bone
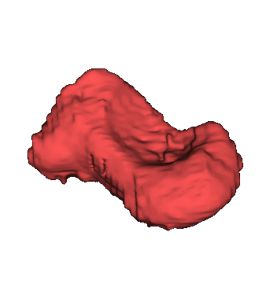
1d) 3D model of scaphoid bone
In a first step the CT data of the patient were analysed to separate bones from the remaining tissue (Virtual environment/ contouring). Next the CT data was transformed into a 3D DICOM model using an Open Source software package. The scaphoid bone was isolated from the complete bone set to generate 3D STL data, a format describing surfaces by triangularization. The STL format is widely used by all kind of 3D printers, also the GeSiM BS3.1.
![Bioprinter 58 2) Scaphoid bone printed from bone cement [1], [2]](https://gesim-bioinstruments-microfluidics.com/wp-content/uploads/2019/07/Printed-bone-1.jpg)
2) Scaphoid bone printed from bone cement [1]
This work is a research project without clinical background. Future research may be focusing on the settlement of osteoblasts or mesenchymal stem cells in the scaffold structure for subsequent incubation and generation of an artificial “living” bone.
We thank the BMWi for funding this work (AiF-ZIM program, project number KF2891602).
References:
[1] T. Ahlfeld, T. Köhler, Ch. Czichy, A. Lode, M. Gelinsky: A Methylcellulose Hydrogel as Support for 3D Plotting of Complex Shaped Calcium Phosphate Scaffolds. Gels 2018, 4, 68 (14 pages)Highly Customized and Affordable Implants by a new Hybrid 3D Printing Technology
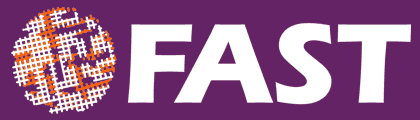
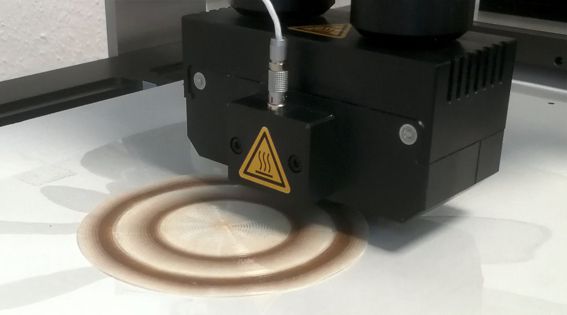
Printing of PEOT/PBT mixture with varying ratio
GeSiM has been engaged for the development of high-performance syringe extruders for printing of polymer gradients within a single AM step. We developed a specialized mixing device to combine two separate HT syringe extruders. The new mixer fits onto the BioScaffold printer BS5.1.
Further GeSiM adapts the NADIR plasma pen onboard of the BioScaffold printer, it now synchronizes with all other BS tools. The plasma pen is a highly flexible tool to modify surfaces layer-by-layer during the 3D print. Depending on its configuration the plasma pen sterilizes, activates surfaces or deposits reactants. This atmospheric plasma jet is realised starting from the unique design developed by Nadir S.r.l. (Patent no. WO / 2015/071746) that ensures an efficient, cold and clean plasma for the surface treatment of the most delicate and heat sensitive materials. Nadir plasma is based on a 27MHz RF (radio frequency) generation system that allow to obtain one of the coldest plasma present in the market, able to preserve a high efficiency in the desired surface treatment of materials
In particular, Nadir torch allows:.
- The surface etching to increase the roughness at nanoscale and to expose the fillers to the surface
- The coating with nucleophilic groups
- The coating with electrophilic groups
- The control of the surface wettability.
Materials for Bioprinting
Several partners developed new biopolymers and fillers. New materials e.g. to mimick artificial bones are now commercially available:
- PEOT/PBT polymer composites (master batches) (Polyvation BV, The Netherlands)
- Fillers as drug delivery systems for bioactive molecules (Prolabin & Tefarn S.r.l., Italy
- Polymer compounds with active and bioactive properties (Nadir S.r.l., Italy)
- Dosable Reduced Graphene Oxide (Abalonyx AS, Norway)
For more information please download the FAST brochure.
The FAST project is scheduled to run for four years from 1st December 2015 to 30th November 2019 with a budget of 4,9 MEUR, which is funded by the European Union under the H2020 Framework Programme for Research and Innovation.
FAST project partners:
- Abalonyx AS, Norway
- Fraunhofer Institute for Surface Engineering and Thin Films IST, Germany
- Fundacion Tecnalia Research & Innovation, Spain
- GeSiM Gesellschaft für Silizium-Mikrosysteme mbH, Germany
- Maastricht University, The Netherlands
- Nadir S. r. l., Italy
- Polyvation BV, The Netherlands
- Prolabin & Tefarm S. r. l., Italy
Press contact via the project coordinators:
Dr. Lorenzo Moroni, Maastricht University,
Dr. Alessandro Patelli, Nadir S.r.l.